Spatial Biology Education
Unlocking the secrets of spatial biology: a journey towards understanding and improving immunotherapy outcomes
Posted on:
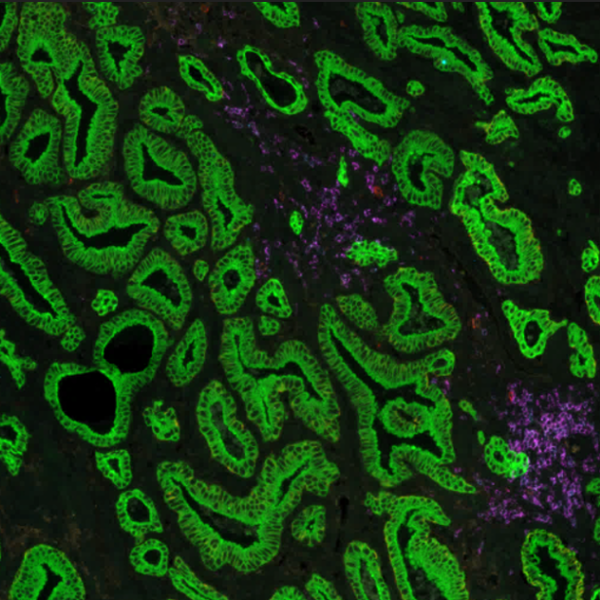
Our bodies comprise billions of complex cells and tissues that keep us alive and functioning. Every single cell in our body is connected in some way to an elaborate network that helps us regulate our physiology. That is why studying the spatial environment of organisms remains one of the most important fields of research today. However, there are still several obstacles to the widespread use of spatial biology techniques. In particular, the level of complexity and the accessibility of learning opportunities can be in the way of gaining a better understanding of when and how to use those tools.
The use of biomarker screening can support improvements in the outcomes of biopharma immuno-oncology studies
Patient identification for optimal treatment has become possible due to the surge of predictive biomarker signatures, which can serve as powerful tools to determine the likelihood of response, or lack of response, to a particular therapy. Biomarker signatures enable the stratification of patient populations, improving therapy response rates in clinical trials, and therefore, they hold considerable potential to optimize both biopharma investment as well as patient outcomes.
Identifying biomarkers with good predictive value requires phenotyping tissue samples of different patient populations and disease states. Gaining insights into the spatial immune environment of tumors is necessary for a thorough understanding of molecular changes that govern disease progression and impact immunotherapy response rates across different populations. Biopharma researchers require versatile tools that offer high-plex analysis capabilities, high-throughput automation, and high reproducibility to fulfill their translational needs. The use of biomarkers in research and clinical studies has grown exponentially in recent years (Figure 1). Since the human genome was sequenced, the use of biomarkers as inclusion or exclusion criteria for enrolling patients in clinical research has substantially expanded1. Some studies have documented the significant impact that biomarker selection can have on regulatory approval rates, demonstrating that a likelihood of a successful transition from Phase I up to Approval is 26% when performed with biomarker selection versus 8.4% without. It is clear that biomarker selection provides a critical tool for improving the efficacy and speed of clinical research.
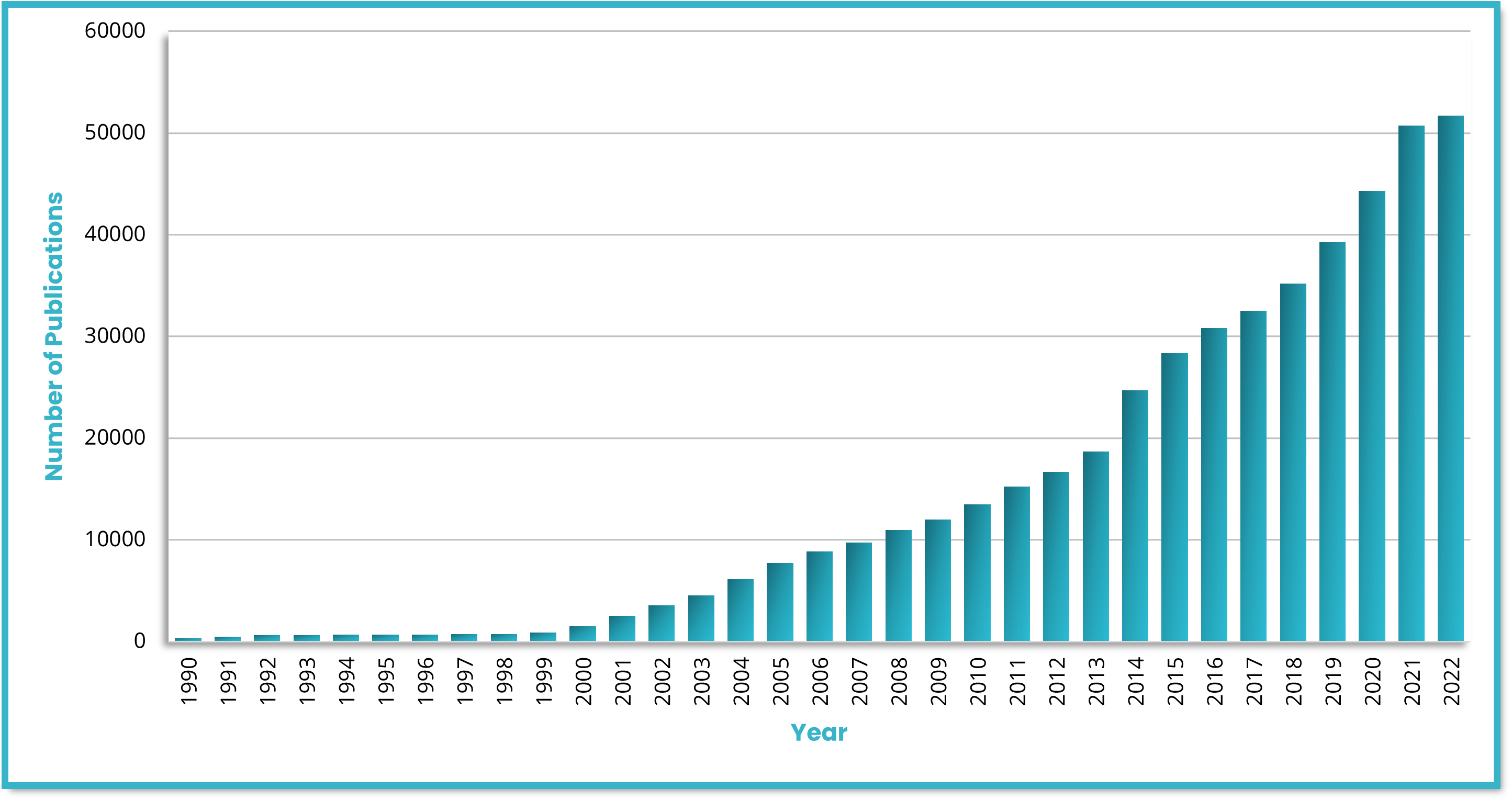
Multiplex immunofluorescence (mIF) shows promising value in identifying tissue-based biomarker signatures
Conventional immunohistochemistry (IHC) is frequently employed as a diagnostic method; however, it has some drawbacks, including the ability to identify only one marker per tissue segment. Multiplex immunofluorescence (mIF) has been developed in response to the growing demand for better techniques2. mIF allows detailed analysis of multiple markers on the same tissue slide preserving spatial context, providing paradigm-shifting insights into immuno-oncology, and revealing the molecular mechanisms underlying cellular heterogeneity within the tumor microenvironment (TME) (Figure 2).
As mIF enables comprehensive interpretation of biomarker distribution, it can answer essential questions about the pathophysiology of many disorders and reveal prognostic biomarkers and novel therapeutic targets. Recent studies demonstrate this potential: Feng et al. showed that multiparametric immune profiling might be useful for stratifying patients with oral squamous cell cancer (OSCC) for clinical trials using mIF3. Johnson et al. performed spatial profiling of PD-1/PD-L1 interaction in metastatic melanoma patients and described the identification of biomarker signatures that correlate with clinical outcomes4. Examples of such findings provide great information about cell composition and spatial arrangements and open new avenues for clinical practice.
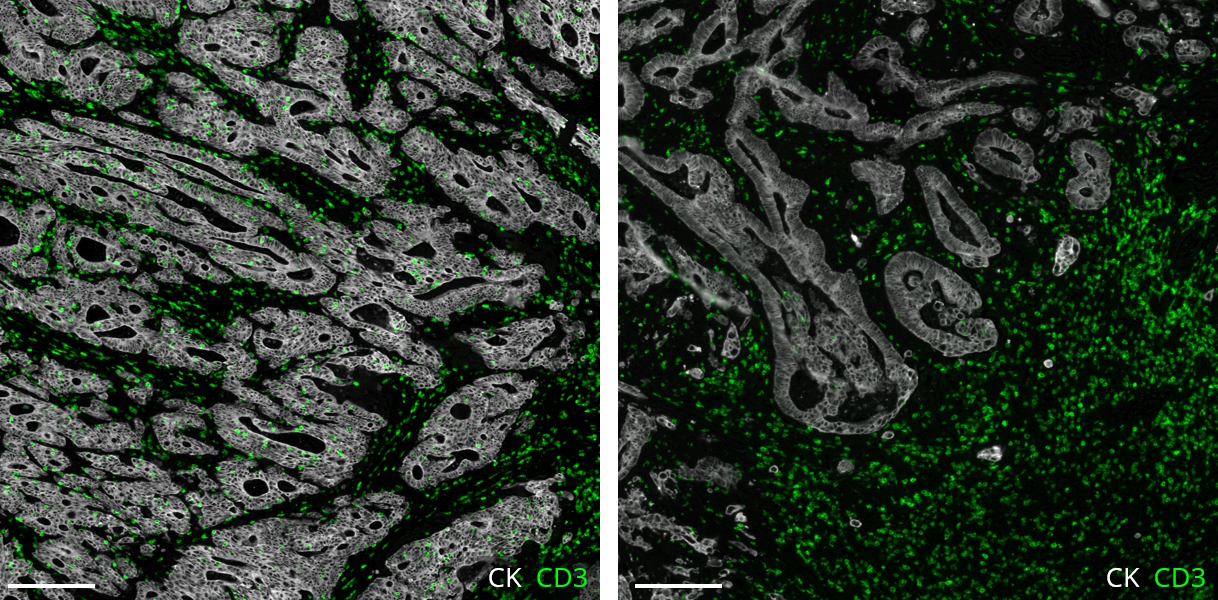
Future directions to take personalized immunotherapy to the next level
In a recent publication, The Society for Immunotherapy of Cancer (SITC) crafted a practical checklist to help researchers gauge the possible impact on patients and the immunotherapy field of phase III trials. As phase III trials for combination immunotherapies advance, authors also indicated numerous directions to maximize their potential value5. That includes studying the efficacy of the short treatments to define a benchmark at which treatments can be deemed successful. In addition, trials should include a comparison of agents administered consecutively to help determine if the effects are truly independent. Identifying, validating, and integrating biomarkers to assess how a patient will respond to treatment are priorities for combination therapy trials that lie ahead5. To make a stride forward, it is also essential to leverage the right technology to accelerate the studies. Molecular profiles obtained through mIF help identify predictive biomarkers and pave the way for new areas of drug discovery. This kind of evaluation could be used in clinical decision-making, adapting well into a routine pathologist’s workflow and, eventually, enabling the tailoring of immunotherapy drugs for suitable patients (Figure 3).
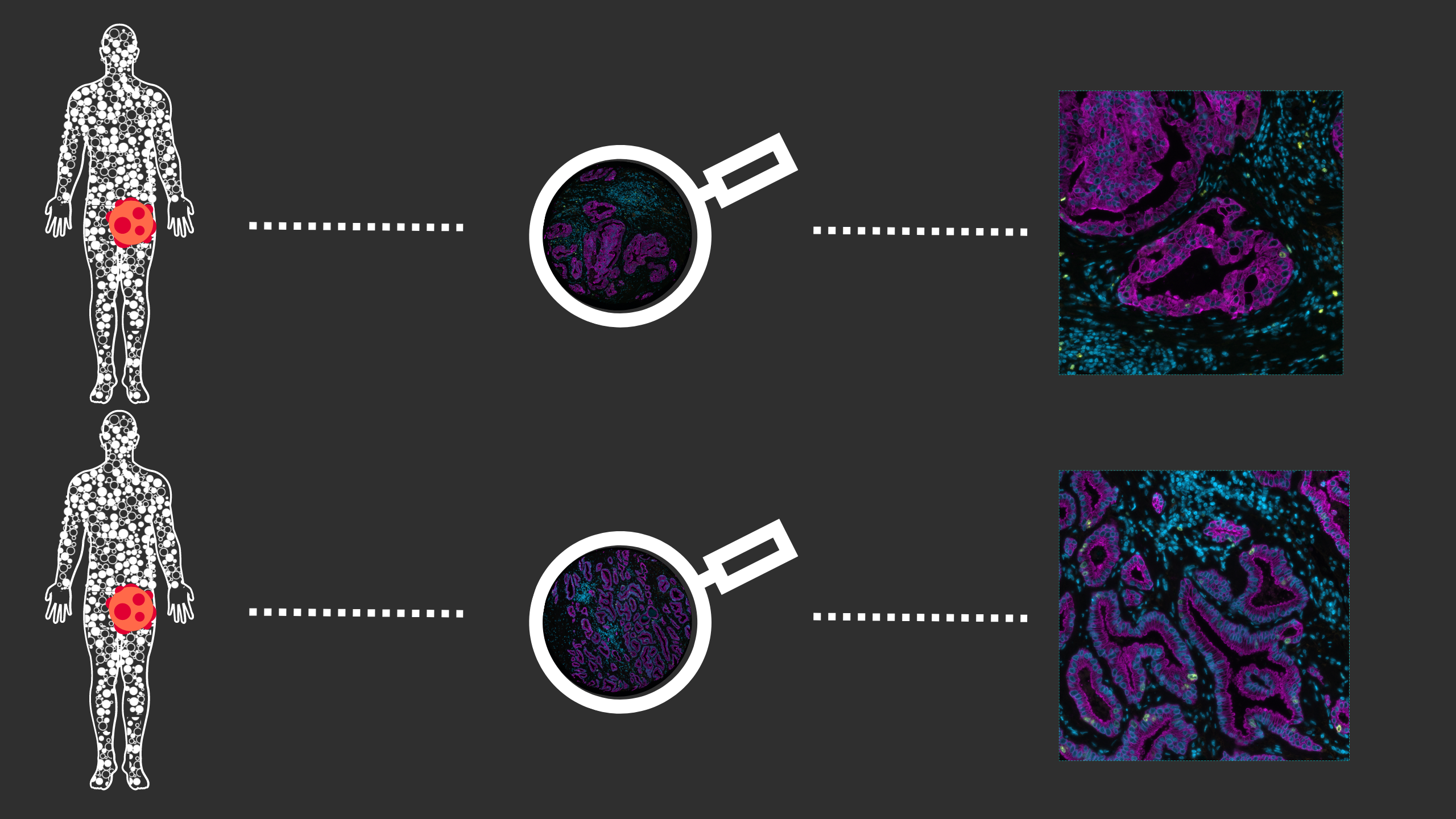
References:
- Thomas D et al. Clinical Development Success Rates 2006-2015; Bio Industry Analysis. 2016.
- Tan WC et al. Overview of multiplex immunohistochemistry/immunofluorescence techniques in the era of cancer immunotherapy. Cancer Commun (Lond). 2020; 40(4):135-153. doi: 10.1002/cac2.12023.
- Feng Z et al. Multiparametric immune profiling in HPV– oral squamous cell cancer. JCI Insight. 2017; 2(14):e93652.doi: 10.1172/jci.insight.93652.
- Johnson D et al. Quantitative Spatial Profiling of PD-1/PD-L1 Interaction and HLA-DR/IDO-1 Predicts Improved Outcomes of Anti–PD-1 Therapies in Metastatic Melanoma. Clin Cancer Res. 2018; 24(21):5250-5260. doi: 10.1158/1078-0432.CCR-18-0309.
- Atkins M et al. Maximizing the value of phase III trials in immuno-oncology: A checklist from the Society for Immunotherapy of Cancer (SITC). J Immunother Cancer. 2022; 10(9): e005413. doi: 10.1136/jitc-2022-005413.