Spatial Biology Education
Assay development: how to overcome the challenges of TSA-based mIHC assays
Posted on:
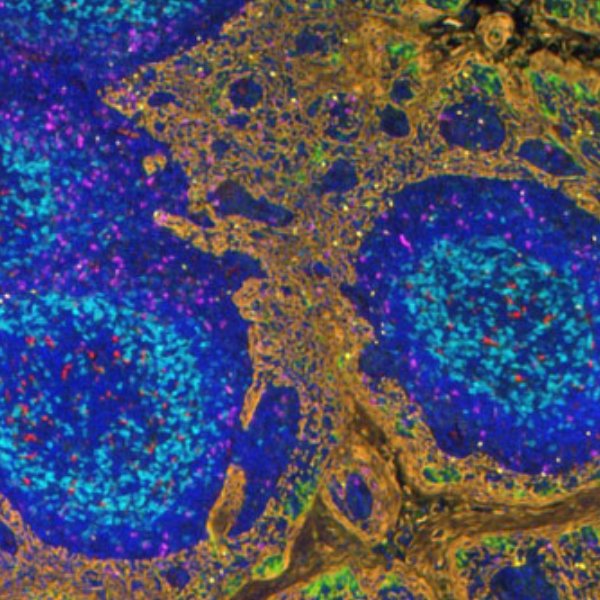
What value do multiplex assays bring to cancer research?
Understanding how the immune system interacts with tumor cells is crucial when developing immunotherapies for cancer treatment. This class of therapies uses the human immune system to combat diseases. However, the clinical efficacy of specific immunotherapies is limited to a subgroup of patients. New strategies to increase the effectiveness of immunotherapies and improve the identification of patients that will benefit from a particular therapy are urgently needed. Research in this field hinges on identifying specific and predictive biomarkers for patient stratification and an improved understanding of the tumor microenvironment (TME).
Multiplex immunohistochemistry (mIHC) is a powerful and proven tool to study the tumor immune context. With mIHC, researchers can concurrently identify multiple epitopes and obtain information about spatial relationships between various cell types in distinct locations. Using spatial profiling for critical tumor-immune pathways can improve the classification of cancer patients for immunotherapy1 and increase the therapy’s efficacy in clinical settings.
What are TSA-based mIHC assays?
Under the umbrella of mIHC, various methods can be used to obtain multiplexed data. Tyramide Signal Amplification (TSA) is an mIHC technique designed to increase the detection of low-abundance targets. TSA can be integrated into conventional immunohistochemistry (IHC) protocols to achieve multiplexed proteomic data or to improve assay precision and sensitivity2.
Unlike conventional chromogenic IHC, where Horse Radish Peroxidase (HRP) converts the diaminobenzidine (DAB) substrate into a brown precipitate in the vicinity of the protein of interest, TSA-based IHC follows a different approach to produce a fluorescent signal. In this case, the HRP will convert a fluorescently-labeled tyramide substrate into a reactive form that covalently binds to tyrosine residues on and near the protein of interest3.
Thanks to the covalent bonds created, the target proteins in a TSA assay are stained sequentially, washing away the antibodies between cycles while keeping the labeled tyramide in place, and all markers can be detected simultaneously at the end of the assay. For each marker, the process is as follows: firstly, the primary antibody and the HRP-conjugated secondary antibody are incubated. Next, the TSA substrate is incubated and covalently binds in the neighborhoods of the protein of interest. Finally, the antibodies are eluted, leaving only the bound TSA reagent behind. The process can be repeated up to seven more times before the tissue is imaged with a fluorescent microscope or a spectral microscope for higher plex assays.
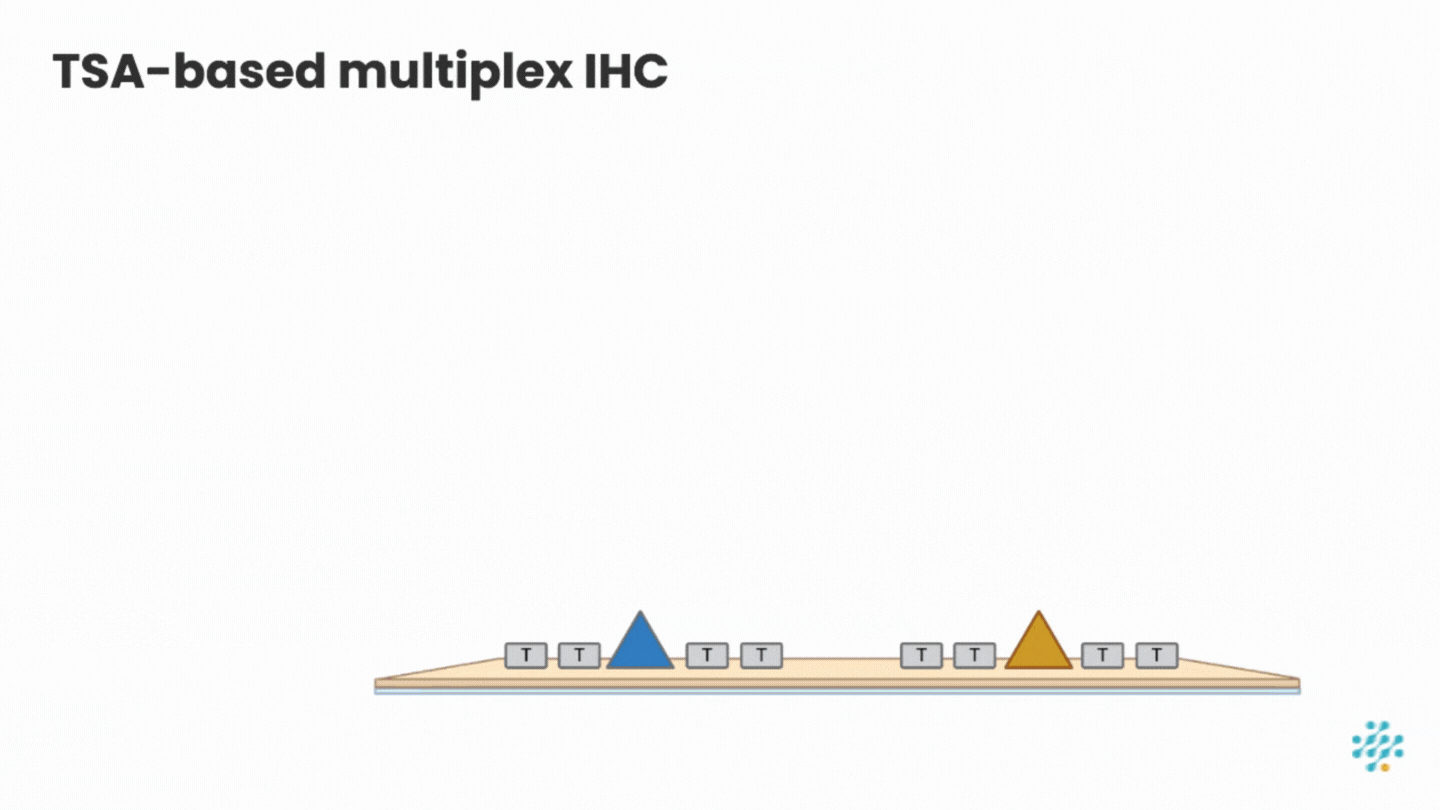
What makes TSA-based mIHC assays challenging?
TSA-based assays can cause researchers many frustrations:
- the high number of manual steps that need to be carefully performed;
- the long protocol duration times;
- the high consumption of TSA reagents.
These challenges are compounded by the complex optimization process requiring multiple cycles before an assay is ready to be used.
How can automation be used to tackle the challenges of TSA assays?
Automation can be integrated into workflows to tackle the main challenges of TSA-based assays. It reduces the amount of time spent at the bench and the quality and reproducibility of the data generated.
LabSat® provides a perfect platform for TSA-based assays on small cohorts of samples. Recently it has been used to investigate the effect of the anti-OPN antibody therapy on immune cell infiltration in the infarct core post-ischemic stroke4. LabSat® is an automated benchtop tissue stainer that enables the automation of IHC and IF assays using off-the-shelf reagents for reproducible and high-quality data. A full 6-plex TSA staining can be run in just four and a half hours with LabSat®.
After the tissue sample is dewaxed, it is loaded onto LabSat®. Antigen retrieval, TSA staining cycles, and elution are automatically performed on the platform. Up to 3-plex, the full staining will be automated. From 4 to 6-plex, a brief pause in the middle of the staining protocol will allow the user to swap the used reagents for the reagents required in the final cycles. Finally, the slide can be retrieved, mounted, and imaged.
Assay automation with LabSat® results in:
- high signal-to-background ratio;
- uniform signal across the whole tissue section (no gradient);
- efficient antibody elution (over 99% for all tested markers);
- high preservation of tissue morphology and epitopes;
- reproducible results;
- transferability of optimized protocols from tonsil to tumoral tissue (you can learn more here).
TSA assays are rapidly and easily optimized on LabSat® before transferring them onto high-throughput instruments.
When working with large cohorts, a single slide stainer will not be sufficient, and users will require the use of high-throughput instruments. Running TSA-based assays on these instruments allows users to generate quality tissue stainings across many samples. However robust mIHC assay optimization is required before large cohorts can be processed.
High-throughput instruments require many optimization cycles before an assay is ready for use on experimental samples. Additionally, high-throughput platforms require large dead volumes of reagents even when running with just a few samples, as is the case during assay optimization. Therefore, optimization performed directly on high throughput instruments can cause the waste of large amounts of TSA reagents.
LabSat® perfectly fills this gap in the workflow of high-throughput TSA assays. The short assay time on LabSat® can be leveraged to rapidly develop and optimize TSA assays to be run on high-throughput instruments. The technical note linked below describes the steps taken to optimize a 6-plex assay on LabSat® and its subsequent, direct transfer to Leica Bond RX.
Download our Technical Note “Multiplex assays easily optimized on LabSat® and directly transferred to high-throughput instruments” and learn how LabSat® has been used to support users in achieving:
- faster multiplex panel development;
- direct transferability of essential staining and imaging parameters to high-throughput instruments;
- reduced turnaround times (from days to minutes) while leaving high-throughput platforms available for high-throughput experiments.
The value of multiplex data for cancer research and biomarker identification is expanding
Multiplex data is of growing importance to support cancer research and biomarker discovery and to inform the development of new cancer therapies and other clinical applications. A simple way to transform classical IHC assays into multiplex IHC assays is to integrate TSA-based protocols into the workflow. In addition to enabling multiplex assays, TSA-based methods improve the sensitivity and robustness of the generated data. Unfortunately, working with TSA reagents can be long, complex, and resource intensive. Integrating LabSat® automation into the TSA workflow will support the adoption of multiplex assays in any laboratory. In addition, laboratories working with high-throughput instruments for processing large sample cohorts can also benefit from the support provided by LabSat® during their assay development and optimization phases.
References
- Johnson D. et al. Quantitative Spatial Profiling of PD-1/PD-L1 Interaction and HLA-DR/IDO-1 Predicts Improved Outcomes of Anti-PD-1 Therapies in Metastatic Melanoma. Clin Cancer Res. 2018; 24(21):5250-5260.doi: 10.1158/1078-0432.
- Stack E. et al. Multiplexed immunohistochemistry, imaging, and quantitation: a review, with an assessment of Tyramide signal amplification, multispectral imaging and multiplex analysis. Methods. 2014; 70(1):46-58.doi:10.1016/j.ymeth.2014.08.016.
- Faget L. and Hnasko T. Tyramide Signal Amplification for Immunofluorescent Enhancement. Part of the Methods in Molecular Biology book series (MIMB, volume 1318).
- Spizer D. et al. Profiling the neurovascular unit unveils detrimental effects of osteopontin on the blood-brain barrier in acute ischemic stroke. Acta Neuropathol. 2022; 144(2):305-337. doi: 10.1007/s00401-022-02452-1.